The University of St. Thomas School of Engineering has formed the Center for Optimized Autonomy and Control (C4OA&C). The mission of this organization is to provide innovative applied learning opportunities for students in engineering and software disciplines, where students can build operational systems, deploy them on focused use cases and engage responsibly with community efforts (both locally and globally) to create technology solutions for the common good.
The center’s first operational system focuses on precision agriculture. Precision agriculture is a crop-production management concept based on implementing modern information technologies such as GPS (Global Positioning Systems), Remote Sensing Technology and GIS (Geographic Information Systems). Precision agriculture is an integral part of emerging crop management practices characterized as the precise application of agricultural inputs for crop growth considering relevant factors such as
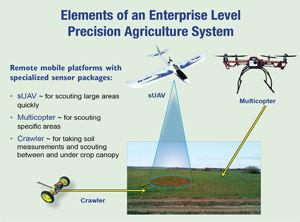
Figure 1: Land-based Sensors for an enterprise-level Precision Agriculture System.
available light, soil, weather and crop management practices. The implementation of this methodology is site-specific and provides a basis for sustainable agriculture with long-term financial and cultural benefits.
The Precision Agriculture Enterprise Concept Project is intended to define, develop, prototype and refine the tools and methodologies necessary to infuse enterprise-level information technology, sophisticated, miniaturized, autonomous remote sensing platforms, and in-situ controls with existing farm machinery to produce best quality agricultural products using a sustainable methodology to revitalize the farmland that produces the product. The project will introduce the technology in a manner that does not require the agronomist to become an IT professional, UAV (Unmanned Airborne Vehicle) pilot, remote sensor operator or data analyst. The goal of this system is to integrate the components shown in Figure 1 at an enterprise-level into a Precision Agriculture System.
Project Goals
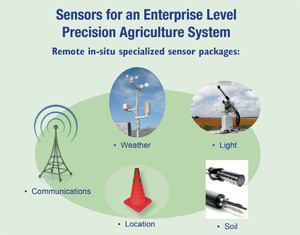
Figure 2: Land-Based Sensors for an enterprise-level Precision Agriculture System.
The goals of this project are to integrate small Unmanned Airborne Vehicles (sUAVs), a multi-rotor helicopter platform (multicopter), multispectral cameras and crawling robots for collecting earth samples and taking measurements below the crop canopy. The required land-based sensors for such a system are shown in Figure 2. The team intends to integrate weather, light and soil information in-situ (in the field) and correlate that data with other Internet-based climate information in order to provide focused tracking of field and crop conditions.
This will lead to the Precision Agriculture System Flow shown in Figure 3. This system combines the use of field readings, sensor re- ports, micro and macro weather conditions and processes the data using a Hadoop/MapReduce cluster for Big Data processing integrating GIS (Geographic Information Systems) data to create command and control outputs for water and chemical distribution.
Near-Term Objectives
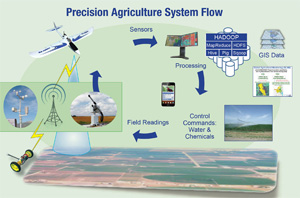
Figure 3: Precision Agriculture System Flow
The UST team is implementing the first-generation, combined testbed, and using this system for practical applications in agriculture. The project will afford our students hands-on experience on how to build, interconnect, operate, and refine these highly integrated systems. Various hardware components have been acquired, a technical road map of goals and sub-projects has been established, and system design documents (including Project Plan and Data Dictionary) have been defined. The team is seeking grant funding to support this work.
Part of this work centers on integrating multispectral cameras into our sUAS and multicopter platforms. Figure 4 shows an example of what this type of camera will produce.
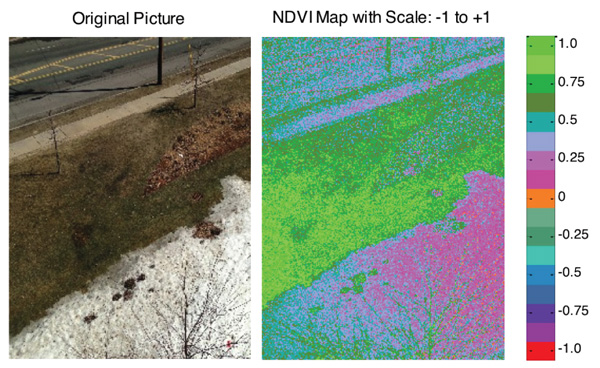
Figure 4: The original picture on the left shows concrete, grass and snow areas. The picture on the right shows the analysis of near-infrared and red light as bright green. These areas have strong photosynthesis and are growing well and unstressed. Magenta areas (snow, concrete, asphalt) indicate no photosynthesis.
Since healthy vegetation strongly reflects near infrared (NIR) light and strongly absorbs red light (less visible light reflection) in the process of photosynthesis, we can use a multispectral camera to collect both visible and NIR light reflected from vegetation and other objects. If we compute the ratio of these light reflections, it will tell us the well-being of the vegetation. This ratio is referred to as Normalized Difference Vegetation Index (NDVI).
The figure shows that healthier (greener) grass areas are brighter green (larger NDVI), while stressed grass areas are indicated by light blue or darker green (smaller NDVI). Magenta areas in the figure have near zero, or negative, NDVI, and they represent concrete road, sidewalk and snow.
International Team
UST researchers have a long history of collaborating with international researchers who are recognized leaders in related research. UST’s work is to combine their results in an engineering platform to provide “best in breed” technologies for our students and faculty to use in their work. This research builds on research with global universities specializing in mono-culture agriculture, and with UST’s established research relationships with faculty at those organizations including:
- UBI, Portugal, Dr. Miguel A. Silivestre, aeronautical engineering;
- University of Minnesota, Dr. David Mulla, Precision Agriculture specialist; and
- University of Minho, Portugal, Dr. Julia C. Viana, polymer scientist.
Bonnie K. Holub, Ph.D.
Holub is a former Honeywell Chair in Global Technology Management in Graduate Programs in Software at the School of Engineering.
Holub is a founder and former CEO of Adventium Labs/Adventium Enterprises, a nonprofit research and development lab. She returned to St. Thomas in 2010. Holub was a tenured faculty member in GPS from 1987 to 2004, during which she founded and directed the Artificial Intelligence/High Performance and Parallel Computing Lab.
Holub holds a Ph.D. in computer science/artificial intelligence from the University of Minnesota. In 2009 she was named the Distinguished Alumnus of the Computer and Engineering Department at the University of Minnesota, Minneapolis, Minn.
James Ellingson, Ph.D.
Ellingson is assistant professor of mechanical engineering in the School of Engineering.
Ellingson studied mathematics, physics, chemistry and philosophy at Concordia College, Moorhead. His graduate studies at the University of Minnesota focused on CAD/CAM/CAE, electrical engineering and modeling of manufacturing processes.
His industrial career includes three years as a member of 3M’s advanced automation group, five years with Boston Scientific’s Vascular Intervention division and one year developing drop on-demand printing and machine vision inspection systems at PPSI.
Ellingson’s broad-based, interdisciplinary approach allows for the creation of appropriate solutions to real-world challenges.
Christopher Greene, Ph.D.
Greene is associate professor and program director of electrical engineering in the School of Engineering.
Greene’s industry experiences have included research in controlling aircraft, spacecraft and advanced navigation system development. He has worked for Honeywell’s Systems and Research Center and later their Avionics Division. Following a brief period at Diversified Technology Systems, where he directed the development of automated semiconductor-processing equipment, he worked for Horton, Inc. (later known as Nexen Group Inc.), where he became product manager for all its industrial products.
His interests include applying PC-based control solutions to industrial control problems. He teaches various electrical engineering courses, including control systems and automation, signals and systems, and electronics.
Scott Morgan, A.S.
Morgan is senior design consultant in the School of Engineering.
Morgan’s support to the UST School of Engineering began in 2004 by integrating a microvideo broadcast system to a STEPS airplane and demonstrating the value of airborne imagery to the students. Following his retirement from industry, Morgan has been a mentor to senior design students.
Before joining UST, Morgan was a Senior Staff Systems Engineer, at Lockheed Martin. His work there includes the design and manufacture of the interior for the Royal Jordanian VIP L-1011, design and manufacture of sensors and communications systems for the USAF BIG SAFARI Program, and conceiving and developing Lockheed Martin’s small unmanned aircraft and control system capability. Other experience includes 20 years in two components of the U.S. military’s Special Operation Forces.
Chih Lai, Ph.D.
Lai is associate professor in Graduate Programs in Software at the School of Engineering.
Lai has taught courses in data mining, multimedia information retrieval, real-time systems and software engineering. In 2010, Lai also worked as a visiting professor at the Informatics Department of Trier University of Applied Science in Germany. Lai is the 2004 University MAXI Grant recipient.
Previously, Lai was a principal software engineer, working on a next generation aircraft collision avoidance system (ADS-B), of which FAA mandates all aircraft to equip by 2020. Dr. Lai received three U.S. patents and three European patents, all related to aircraft collision avoidance algorithms. He also works with Medtronic on patient monitoring and movement analysis.
From Exemplars, a publication of the Grants and Research Office.